Engineering Innovation
Research
See Our Work
Mizzou Engineers are exploring new possibilities in our innovative centers and labs.
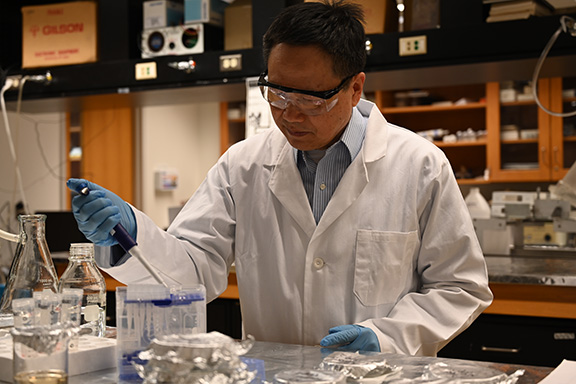
Find Support
Meet our Engineering Research Administration staff and learn how they support faculty research.
Research News Feed
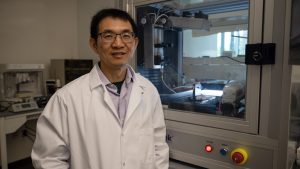
June 17, 2025
Mizzou Engineering researcher takes on tooth loss
A novel, bio-inspired treatment for periodontitis could help patients keep their natural teeth, avoiding painful and expensive implants.
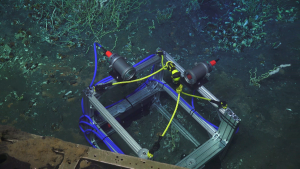
June 10, 2025
Snapshots of the deep shed light on hydrocarbon seeps
Mizzou Engineers have developed a low-cost mobile technology to study naturally occurring gas leaks beneath the ocean’s surface.
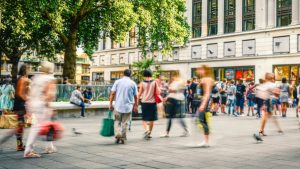
May 21, 2025
Study explores how to use AI to listen to the ‘heartbeat’ of a city
Mizzou researchers develop an artificial intelligence tool that analyzes public Instagram posts to aid city safety, services and emergency response.
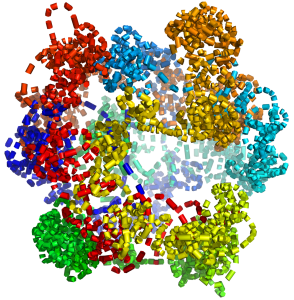
May 28, 2025
New AI tool reveals single-cell structure of chromosomes in 3D
A breakthrough at Mizzou Engineering could help scientists better understand how genes work — and how certain types of cancer develop.